Binder Jetting is an Additive Manufacturing process in which a binding liquid is selectively deposited to join powder material together to form a 3D part.
What is Binder Jetting?
Binder Jetting (BJ) is one of seven Additive Manufacturing techniques in which a binding liquid is selectively deposited to join powder material to form a 3D part. Among the additive manufacturing technologies today, binder jetting is unique because it does not employ heat during the process like others to fuse the material.
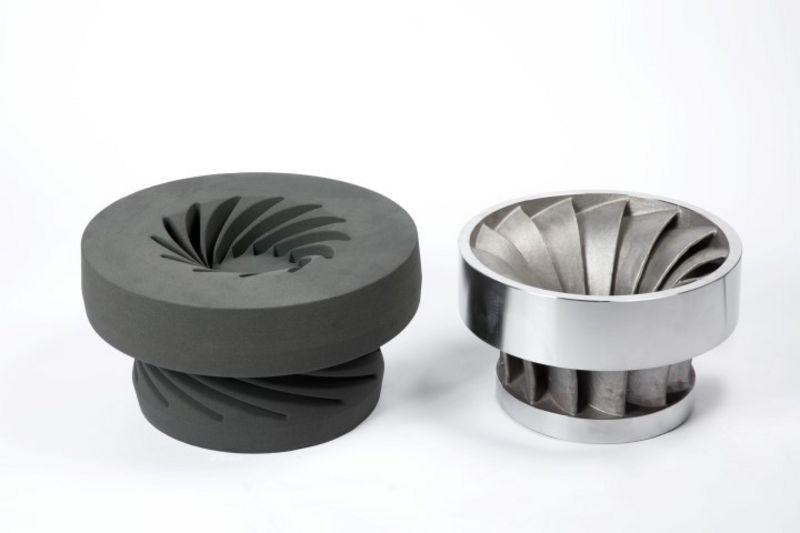
Types of Binder Jetting
It can be classified into the following groups by the binding agent it uses to combine the material to form the object. Binders are critical to a successful BJ process. The type of binder used depends on the type of powder or system that is being used or customer application requirements.
One of the pioneers of this binder technology, Exon uses the following binders.
- Furan Binder
- Silicate Binder
- Phenolic Binder
- Aqueous-Based Binder
How does Binder jetting work?
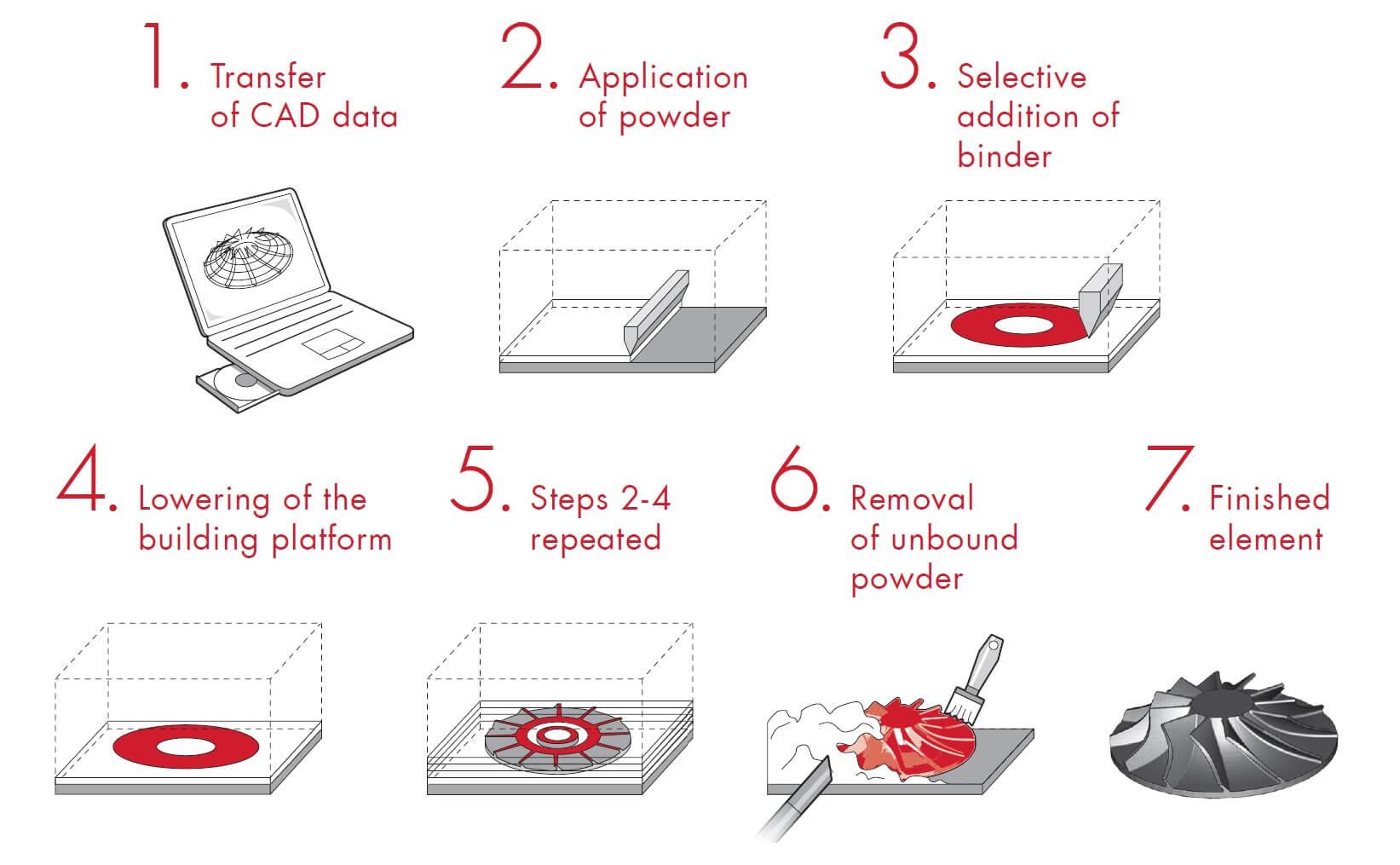
- The powder material is spread over the build platform using a roller
- The print head deposits the binder adhesive on top of the powder where required
- The build platform is lowered by the model’s layer thickness
- Another layer of powder is spread over the previous layer. The object is formed where the powder is bound to the liquid
- The unbound powder remains in position surrounding the object
- The process is repeated until the entire object has been made
Descriptive video of a Binding Jetting process by Voxeljet
Advantages and disadvantages of Binder jetting
Advantages of BJ
- Parts can be made in a range of different colours
- It uses a range of materials: metal, polymers and ceramics
- The process is generally faster than others
- The two-material method allows for many different binder-powder combinations and various mechanical properties.
Disadvantages of BJ
- Not always suitable for structural parts due to the use of binder material
- Additional post-processing can add significant time to the overall process